Research Overview
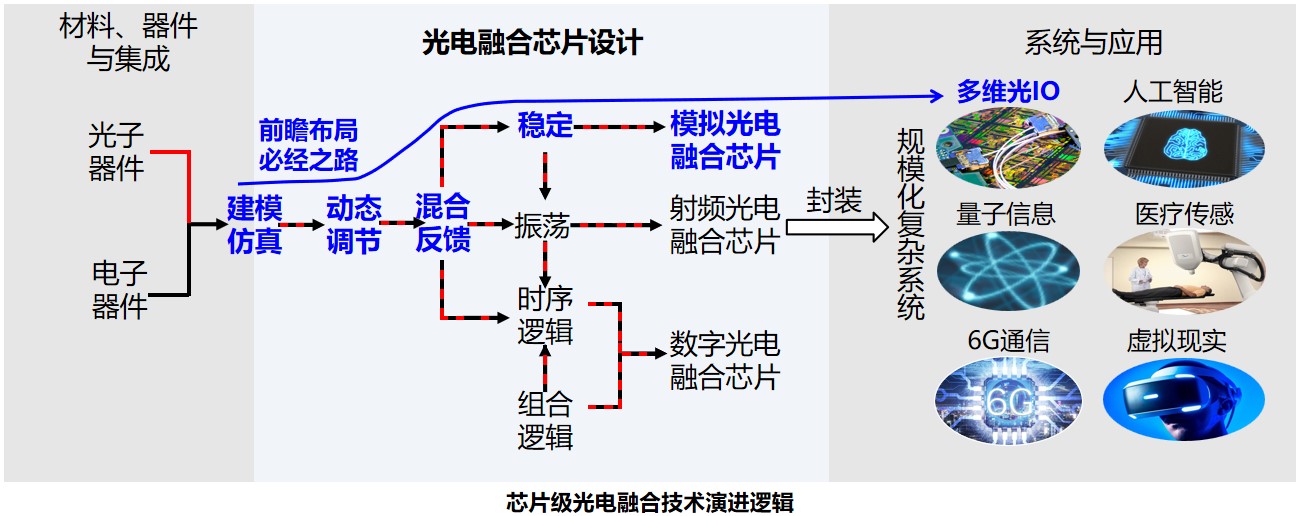
We are interested in Fast, Reliable, Energy-Efficient (FREE), and Scalable integrated circuits for manipulation of and interaction between electrons and photons with applications to communication, computing, and sensing. We have proposed the Electronic-Photonic Heterogeneously-converging IC (EPHIC) framework that combines integrated photonics and integrated electronics at the circuit level by leveraging the experiences of electronic integrated circuits. In particular, we are interested in the following fundamental research directions:
- Power, energy, and thermal problems in silicon photonics.
- On-chip hybrid closed-loop photonic parameter stabilization.
- Fundamental design theory and basic IC building blocks.
- AI for EPHIC design automation and EPHIC for AI systems.
- Electronic-phototonic heterogeneously-converging chiplet.
- Ultra-low-loss waveguides and high efficiency optical coupling.
- Miniaturized equipments for photonic parameter measurement.
- Uncertainty characterization and quantification of photonic devices.
Current Research Topics
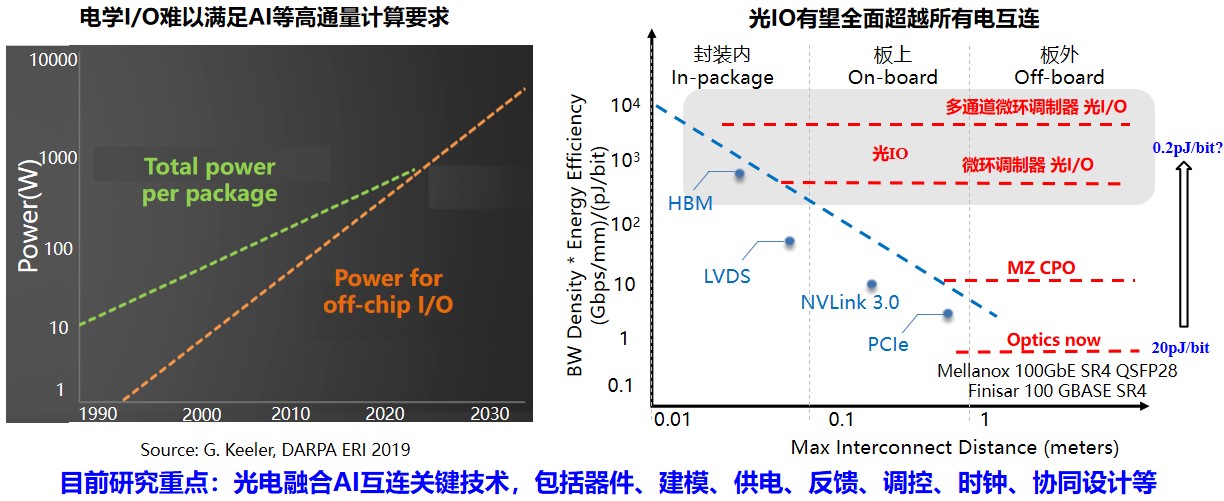
Power and Thermal Management of EPHIC
All active integrated photonic devices, (e.g. laser diode, photodiode, etc.), need power supply. Furthermore, almost all resonator photonic devices
need thermal control to maintain a fixed resonant frequency. Also, thermal tuning is one of the most commonly used methods to control on-chip photonic parameters. At last, the related electronic building blocks require power supplies. Power and thermal control is an indispensable part of EPHIC.
References
[1] M. Tan*, C. Zhan, and W.-H. Ki, “A 4uA quiescent current output-capacitor-free low-dropout regulator with fully differential input stage,” in IEEE International Symposium on Circuits and Systems (ISCAS), Melbourne, Australia, pp. 2457-2460, Jun. 2014.
[2] Yifan Zhang, M. Tan*, “A buck converter using a fully-integrated current-mode dual-path type-III compensator for NB-IoT applications”, in Proc. IEEE Int. Conf. on Integrated Circuits, Technologies and Applications (ICTA), Beijing, China, Jul., 2018
[3] 谭旻,谢子颖 “一种多输出LDO电路以及基于LDO的多电压输出方法” (专利申请号:201810357748.5)
[4] 谭旻,叶开轩 “一种基于数字线性稳压器的多通道电源输出电路及方法” (专利申请号:201910293366.5)
[5] 谭旻,杨超 “一种超低电压LDO电路”(专利申请号:201910139295.3)
On-Chip Stabilization of Photonic Parameters
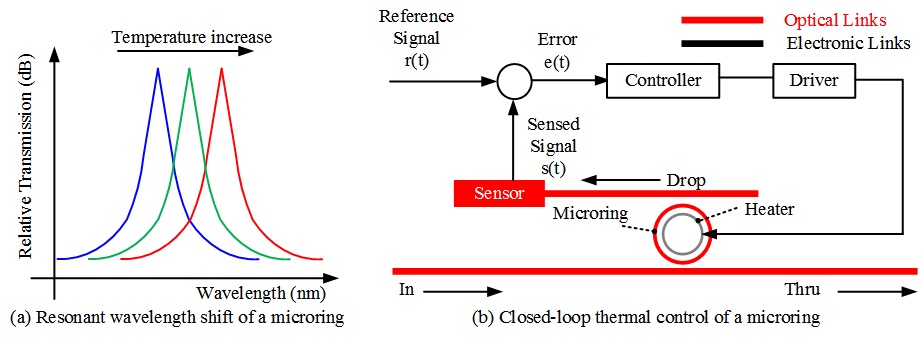
Photonic parameters (e.g. wavelength, phase, etc.) are sensitive to fabrication variations, temperature, cross talk, and so on. Due to both random and systematic variations, it is difficult to resolve this challenge by improving the fabrication process alone [2]. On-chip stabilization of photonic parameters is the key to practice and large-scale integrated photonic systems. On-chip closed-loop electronic-photonic feedback control so far seems to be the only way to resolve this above challenge effectively.
References
[1] Zhicheng Wang, Yu Yu, Xi Xiao, Miaofeng Li, Xuecheng Zou, Dingshan Gao, M. Tan*, “A time-division-multiplexing scheme for simultaneous wavelength locking of multiple silicon micro-rings”, in Proc. IEEE Int. Symp. Circuits Systems (ISCAS), Florence, Italy, May, 2018
[2] Sun, C., et al. "Single-chip microprocessor that communicates directly using light." Nature
528.7583(2015):534.
[3] S. Agarwal, et al. "Wavelength locking of a Si ring modulator using an integrated drop-port OMA monitoring
circuit," in IEEE Journal of Solid-State Circuits, vol. 51, no. 10, pp. 2328-2344, Oct. 2016.
[4] Da Ming, Zhicheng Wang, Lining Zhang, M. Tan*, “A Verilog-A compact model for silicon micro-ring supporting fast thermal-electronic-photonic co-simulation”, in The 10th Int. Conf. on Information Optics and Photonics (CIOP), Beijing, China, Jul., 2018
[5] Yuhang Wang, Da Ming, M. Tan*, “Closed-loop thermal-electronic-photonic co-simulation for a novel Mach-Zehnder modulator bias control scheme ”, in The 11th Int. Conf. on Information Optics and Photonics (CIOP), Xi'an, China, Aug., 2019
Dynamic Power Supply for 5G/IoT/Silicon Photonics
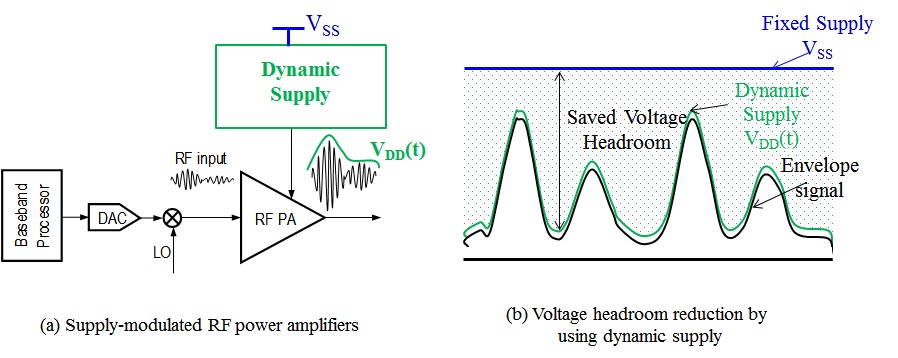
The orthogonal frequency division multiplexing (OFDM), which is the key technology for
5G/NB-IoT wireless
commutations, suffers from the high peak-to-average power (PAPR) ratio [1]. To maintain sufficient linearity
under a
high PAPR, the RF power amplifier (PA) has to use a large power back-off, which degrades the efficiency
significantly. This linearity and efficiency tradeoff problem is widely recognized as one of the key challenges
for 5G/NB-IoT wireless systems. Supply-modulated RF PA [2] is one of the most promising techniques to resolve this
challenge but critically relies on a wide bandwidth high-efficiency supply modulator. Both linear regulator and
switching regulator fail to meet these two requirements simultaneously. The hybrid
supply modulator (HSM), which combines a linear regulator and a switching regulator
in parallel, is a promising solution to achieve both high linearity and high
efficiency. We have proposed several novel HSM architectures [3],[4].
References
[1] T. Jiang and Y. Wu, “An overview: Peak-to-average power ratio reduction
techniques for OFDM signals,” IEEE Trans. Broadcast., vol. 54, no. 2,
pp. 257–268, Feb. 2008.
[2] B. Kim, J. Kim, D. Kim, J. Son, Y. Cho, and B. Park, “Push the envelope:
Design concepts for envelope-tracking power amplifiers,” IEEE Microw.
Mag., vol. 14, no. 3, pp. 68–81, Mar. 2013.
[3] M. Tan and W.-H. Ki, “An efficiency-enhanced hybrid supply modulator with single-capacitor
current-integration control,” IEEE J. Solid-State Circuits (JSSC), vol. 51, no. 2, pp. 533–542, Feb. 2016.
[4] M. Tan and W.-H. Ki, “ A 100MHz hybrid supply modulator with ripple-current-based PWM control ,” IEEE
J. Solid-State Circuits (JSSC), vol. 52, no. 2, pp. 569–578, Feb. 2017.
[5] Yifan Zhang, M. Tan*, “A buck converter using a fully-integrated current-mode dual-path type-III
compensator for NB-IoT applications”, in Proc. IEEE Int. Conf. on Integrated Circuits, Technologies and
Applications (ICTA), Beijing, China, Jul., 2018
Basic IC Building Blocks

We have proposed a number structures for two-stage [1],[2] and three-stage amplifiers
[3]. Furthermore, we have established a
generic model [4] that links the compensation techniques used in two-stage amplifiers to the structures of
three-stage
amplifiers. Our previous results provide a solid foundation for further investigation of amplifiers and other
basic
IC building blocks.
References
[1] M. Tan and W.-H. Ki, “Current-mirror Miller compensation: An improved frequency compensation
technique for two-stage amplifiers,” in International Symposium on VLSI Design, Automation, and Test (VLSI-DAT),
Hsinchu, Taiwan, pp. 312-315, Apr. 2013.
[2] M. Tan and W.-H. Ki, “Split-output miller-compensated two-stage amplifiers,” in IEEE International
Conference of Electron Devices and Solid-state Circuits (EDSSC), Hong Kong, China, Jun. 2013.
[3] M. Tan and W.-H. Ki, “A cascode Miller-compensated three-stage amplifier with local impedance
attenuation for optimized complex-pole control,” IEEE J. Solid-State Circuits (JSSC), vol. 50, no. 2, pp.
440–449, Feb. 2015.
[4] M. Tan and W.-H. Ki, “A generic model for constructing three-stage amplifiers,” in IEEE International
Symposium on Circuits and Systems (ISCAS), Montreal, Canada, May 2016.